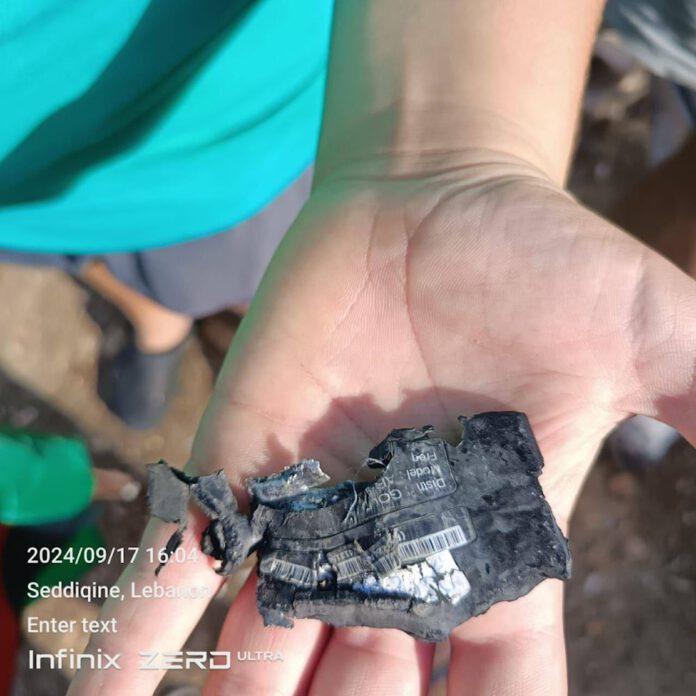
On September 17, thousands of pagers and walkie-talkies were simultaneously detonated across Beirut and several regions in southeastern and northeastern Lebanon, causing widespread devastation, with thousands injured and 12 fatalities.
This alarming event raises critical questions: How can a pager, typically a communication device, be rigged to explode? More importantly, can such unconventional explosives be detected before they are triggered? These questions point directly to the challenges and importance of “Unmanufactured Explosives Detection” in modern security frameworks.
Reports indicate that the Lebanese bombing involving pagers occurred due to the infiltration of the supply chain by terrorist attackers, who implanted a small amount of PETN inside the devices. PETN, a potent explosive, was detonated by overheating the pager’s battery through short-circuiting, resulting in an explosive chain reaction. This “exploding pager” serves as a classic example of an unmanufactured explosive—non-standard, improvised devices that have repeatedly surfaced in bombings across the Middle East, South Asia, and North Africa.
Explosives, broadly speaking, can be categorized into two types. The first are standard explosives, which include military-grade munitions such as artillery shells, bombs, and grenades, produced by industrial enterprises. In contrast, improvised explosive devices (IEDs) are non-standard, handmade explosives, often assembled using everyday materials such as chemical agents and fertilizers. These raw components—nitrates, ammonium salts, hypochlorite, chlorates, perchlorates, urea, and oxidizing agents—are mixed with flammable substances like sulfur, petroleum, or carbon powder. The ease of access to these materials and the simplicity of their production methods pose a serious challenge to global security.
Real-world events underscore the destructive potential of such improvised explosives. The 2013 Boston Marathon bombing, for instance, was carried out using pressure cooker bombs filled with low-cost explosives and packed with shrapnel, including shotgun shells, screws, and bullets. Despite costing less than $100 to assemble, these makeshift devices claimed three lives and injured over 260 others. Such examples highlight the alarming accessibility and lethality of non-manufactured explosives, which have emerged as a common weapon in both fictional narratives and real-world terrorist incidents.
These cases illustrate that the destructive potential of homemade, non-manufactured explosive devices can rival, or in some instances, closely approximate that of industrially manufactured explosives. Certain improvised devices can achieve up to 70% of TNT’s explosive power, making them highly dangerous. While the structure and principles behind manufactured explosives are well understood, allowing for more standardized handling and disposal, non-manufactured explosives pose a greater challenge.
Their complexity stems from the wide variety of materials and methods used in their creation, with different detonation triggers and chemical compositions that are often known only to the bomb maker. This variability makes detecting and neutralizing such devices particularly difficult, as traditional detection systems may not recognize the diverse array of components. Consequently, addressing the threat of non-manufactured explosives requires sophisticated detection techniques and adaptive security measures.
Currently, both domestic and international research institutions have developed various explosion-proof security technologies, with X-ray detection and ion mobility spectrometry being the most commonly used.
X-ray detection technology focuses on identifying the external shape and structural characteristics of explosive devices. By scanning items with X-rays, the technology generates images based on varying degrees of X-ray absorption by different materials, allowing for the identification of potentially dangerous items.
This method is widely employed for screening baggage and parcels in public spaces such as airports, subways, and train stations. For instance, airport security personnel rely on X-ray machines to produce detailed images of the contents of luggage, helping to quickly spot contraband like firearms, knives, or explosives. However, when it comes to liquids, security staff often ask passengers to open containers for manual inspection, as X-ray machines struggle to differentiate between the specific compositions of organic and inorganic substances.
In contrast, ion mobility spectrometry (IMS) is a highly sensitive molecular detection technique that leverages the intrinsic properties of explosives. It detects substances by measuring the migration rates of ions within an electric field. The principle involves sample molecules reacting with reactant ions to form charged product ions—positive or negative—depending on the material.
Typically, drug molecules show high proton affinity, while explosive molecules have high electron affinity, enabling the machine to differentiate between them by altering the electric field direction. IMS technology is commonly deployed in airports, railway stations, logistics hubs, and at large-scale events for explosive detection. For example, during airport security checks, personnel may use a small test paper to wipe a passenger’s luggage or clothing, then analyze it with an IMS device to detect even trace amounts of explosive particles.
Together, X-ray detection and ion mobility spectrometry offer complementary approaches to detecting non-manufactured explosives by examining both external features and internal chemical properties. However, both technologies have limitations. X-ray detection struggles with certain liquids, colloids, and newer or specially formulated explosives, while IMS shows reduced sensitivity when dealing with low-volatility substances or materials with similar chemical properties, and may sometimes fail to detect unstable compounds under certain conditions.
In the international fight against hidden explosives, explosive detection dogs remain a vital tool due to their highly developed sense of smell. These dogs can detect the extremely faint odors of explosives with remarkable sensitivity. To put this into perspective, while humans can detect concentrations as low as one part per million, and advanced chemical analysis methods can detect concentrations as low as one part per billion, a detection dog can identify odors at concentrations as low as one part per hundred billion—10,000 times more sensitive than a human. Even tightly sealed explosive devices emit trace odors, which detection dogs can detect, accurately pinpointing the location of the threat.
However, there are limitations to using explosive detection dogs. Training these dogs takes considerable time (18-24 months) and is costly, with each dog costing approximately $50,000. In practice, dogs can only work for a few hours a day due to fatigue and susceptibility to distractions. This limited endurance poses challenges for large-scale, high-efficiency detection in complex operational environments.
To overcome these limitations, researchers are turning to the field of biological olfaction, which is the complex sensory process that allows animals to detect and identify specific odor molecules. Building on extensive research into olfactory mechanisms, scientists have begun developing artificial olfactory systems with bio-mimetic capabilities, often referred to as “artificial noses.” These systems include electronic noses, bioelectrical noses, and colorimetric noses. However, one major limitation of artificial noses is their inability to detect substances with very low volatility or non-volatile substances, which restricts their effectiveness in explosive detection.
Addressing this challenge, a scientific research team from Xinjiang Institute of Physics and Chemical Technology of the Chinese Academy of Sciences has made significant advances in artificial olfactory systems. Their innovative approach focuses on creating a highly sensitive, rapid, and visual detection system. By developing a hydrogel-based colorimetric artificial olfactory system, the team has managed to convert smells into visible images. This breakthrough offers a promising new solution for detecting otherwise undetectable odors, opening new possibilities for more efficient and reliable explosive detection technologies.
The use of hydrogel in this innovative colorimetric artificial olfactory system leverages its unique properties—a micro-liquid environment combined with solid mechanical characteristics—mimicking the function of the olfactory mucosa found in the nasal cavity. This allows for the adsorption, dissolution, and detection of suspended particles, such as unmanufactured explosives, in the air. The hydrogel is loaded with a colorimetric probe designed to detect specific substances, simulating the function of olfactory receptor proteins, which bind to odor molecules.
The visible image emerges through a sequence of interactions. Initially, the hydrogel physically adsorbs airborne particles of unmanufactured explosives onto its surface. As these particles dissolve within the hydrogel, they react with the embedded reagents in the colorimetric probe, resulting in a distinct color change. This localized color shift creates a visible image that reflects the presence of specific explosive particles.
This colorimetric artificial olfactory system is highly effective in mimicking biological processes. By combining hydrogel arrays with specialized reagents, it replicates the detection and identification mechanisms of olfactory mucous membranes and odor-binding proteins, achieving sensitivity levels as high as one part per trillion. This breakthrough addresses a key limitation of traditional detection technologies such as electronic, bioelectrical, and colorimetric noses, which struggle with low-volatility or non-volatile substances.
Beyond explosives detection, this technology has far-reaching potential applications. Its high sensitivity and adaptability make it valuable in drug detection, environmental monitoring, food safety, and other critical fields where precise identification of trace substances is essential.
Source: XJIPC, nobelprize, Anadolu Agency, Mondoweiss